Primary source information:
Authors: Seohyun Chris Kim, Lijun Zhou, Wen Zhang, Derek K. O’Flaherty, Valeria Rondo-Brovetto, and Jack W. Szostak
Journal: J. Am. Chem. Soc. 2020, 142, 2317−2326
Cover image: Johan Swanepoel, Shutterstock
Modern Earth is teeming with life, but that has not always been the case. The Earth formed about 4.5 billion years ago, and at that time the planet was hot and uninhabitable. But after a relatively short period of cooling, geological evidence suggests that the first life appeared on Earth. How did that happen?!?! The origin of life is still one of the biggest mysteries facing the scientific community.
A leading hypothesis for the origin of life is called “RNA world”. In the RNA world, RNA molecules act as both storage for genetic information and as functional enzymes. In contrast, modern life uses DNA to store genetic information, and protein enzymes to perform most functions. Biology classes often teach that RNA is only a carrier of information between DNA and protein. But that’s not true! Many functional RNA enzymes have been discovered (for instance, the ribosome). The dual ability to store genetic information and have enzymatic function suggests that RNA could have played a central role during the origin of life. If RNA molecules can form spontaneously from a mixture of building blocks, that would be a major first step toward life!
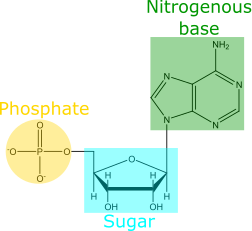
In their recent paper, Kim et al. seek to understand how an RNA molecule can form from its nucleotide building blocks. (Remember: RNA is a chain of nucleotide building blocks. Each nucleotide consists of a sugar, a nitrogenous base, and a phosphate [Fig 1]). But these researchers noticed a problem: the chemical pathways to synthesize nucleotides on early Earth would not simply give the building blocks of RNA. Instead, these chemical pathways can yield at least 3 different versions of nucleotides [Fig 2]: ribonucleotides (the version found in RNA), deoxyribonucleotides (the version found in DNA), and arabinonucleotides. (Note: These 3 versions of nucleotides each have a different sugar component. But each version still has 4 options for nitrogenous base. So ribo-G, deoxy-G, and ara-G are all nucleotides that have different sugar components, but the same nitrogenous base). If the early Earth provided a mixture of these different nucleotide versions, why is modern RNA composed exclusively of ribonucleotides? How did pre-biological chemistry of the early Earth achieve this purity?
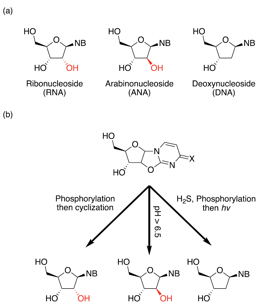
To address these questions, the researchers set out to measure how long it takes to add each nucleotide version to a growing RNA chain. Ribonucleotides, deoxyribonucleotides, and arabinonucleotides were separately reacted to elongate an RNA chain. Once the nucleotides became incorporated into the chain, those chains were analyzed via gel electrophoresis. Gel electrophoresis separates RNA molecules based on length, and so the researchers were able to separate the initial RNA chains from the extended RNA chains. After separation, the concentration of these RNAs was measured with the help of a fluorescent marker embedded in the chain. This data allowed the researchers to calculate the rate at which different nucleotides were added [Fig 3]. These data suggest that addition of a ribonucleotide to a growing RNA chain is much faster than addition of the other two versions of nucleotides. This begins to explain how pure RNA could form: the RNA nucleotides get added to a growing chain faster than deoxyribo- or arabino- versions.
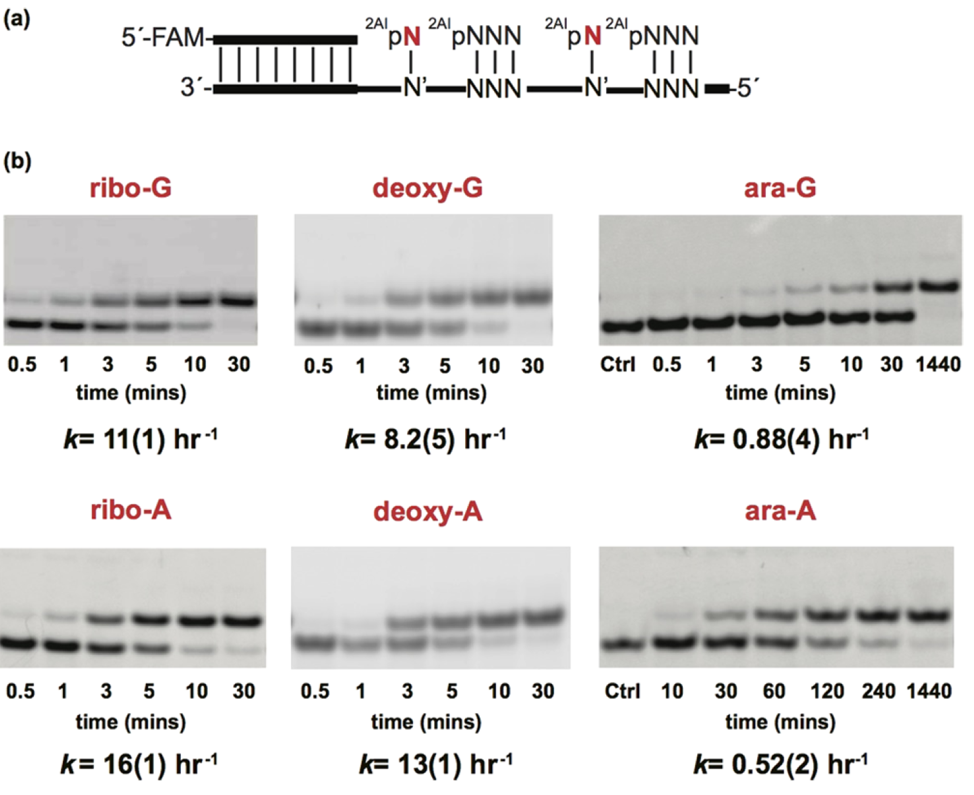
Next, the researchers asked how an incorporated deoxy- or arabinonucleotide affects the rate of addition of subsequent nucleotides. Using similar methods to their last experiment, they find that if a deoxyribonucleotide does get added to the chain, then the addition of the next nucleotide is significantly slower [Fig 4]! This means that the deoxyribonucleotide hinders chain elongation. But even more shocking, if an arabinonulceotide gets added, then the addition of the next nucleotide is completely prevented! This is a huge problem; long chain lengths are needed for RNA to store information and develop enzymatic functions. If an arabinonucleotide prevents chains from growing to long lengths, its incorporation in RNA polymers would be a major barrier for the origin of life.
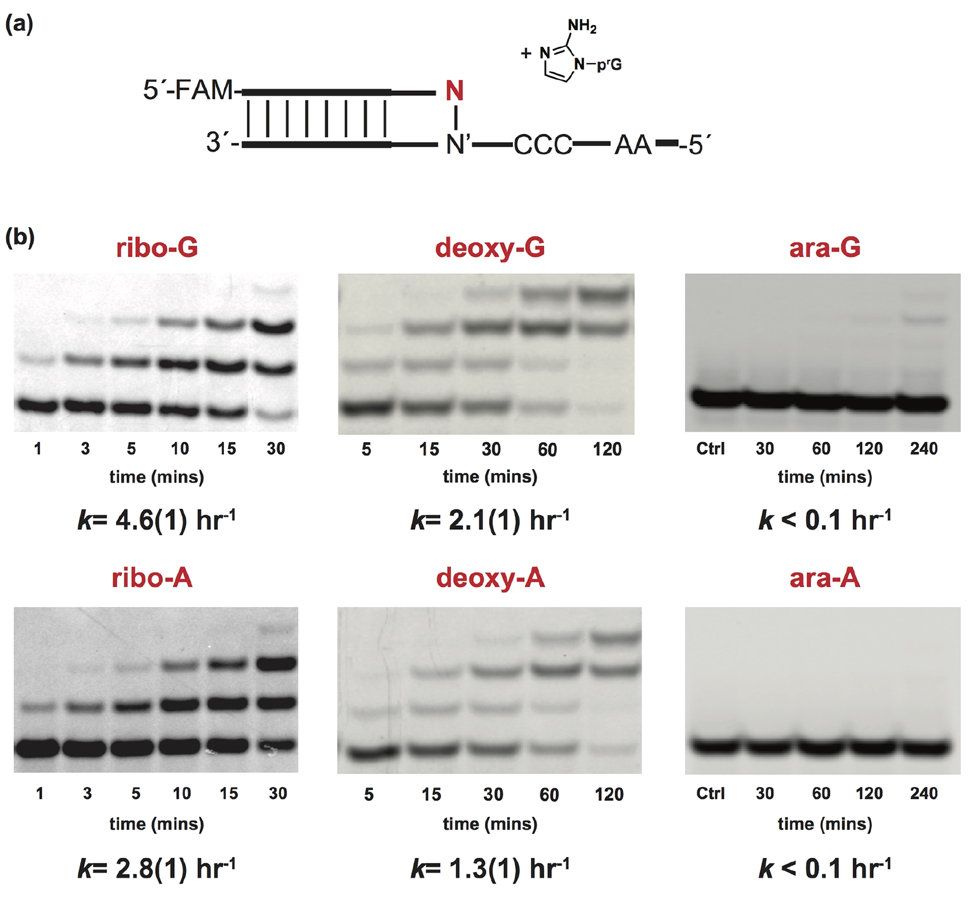
Figure 4: Having a deoxyribonucleotide at the end of an RNA chain significantly slows down elongation of the chain, while arabinonucleotides completely prevent further elongation. a) Schematic representation of an RNA chain that ends in one of the six nucleotides shown. b) Gel electrophoresis shows separation of initial RNA chains from the extended RNA chains. The earlier accumulation of the upper bands (extended RNA) for the ribonucleotides indicates that these reactions are happening faster.
But remember, arabinonucleotides get incorporated into RNA chains more slowly than ribonucleotides. So there is hope: if ribonucleotides react with the growing chain before the arabinonucleotides, the chain could continue to grow long. The researchers test this in a competition experiment [Fig 5]. A mixture of ribonucleotides and arabinonucleotides are allowed to react together with an RNA chain. Both ribo and arabino versions have the same opportunity to incorporate into the chain. If a ribonucleotide gets incorporated, that’s good; chain elongation can continue. If an arabinonucleotide gets incorporated, that’s bad: no more chain elongation can occur. The results of the experiment show that, indeed, barely any arabinonucleotides get added to the RNA chain. Only the ribonucleotides are incorporated! Because ribonucleotides can add onto the RNA chain more quickly than arabinonucleotides, the ribo- version is significantly more likely to be incorporated, and chain termination is not problematic.
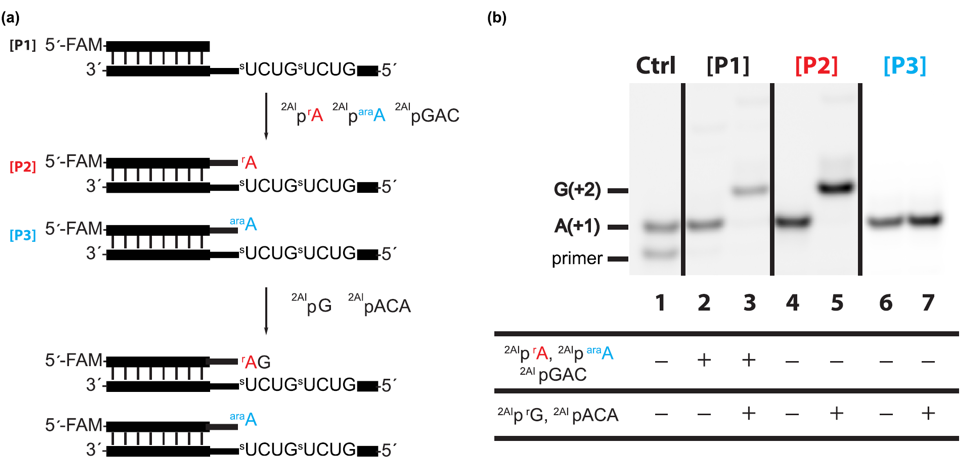
These results suggest that it is plausible for pure RNA to emerge from a mixture of nucleotide building blocks, because the ribonucleotides get incorporated into RNA chains more rapidly than the other nucleotide versions. This is an exciting finding that supports the RNA world hypothesis for the origin of life! But there’s still so much to be learned about the chemistry that led to life’s origin, so keep your eyes peeled for future updates!