Primary Source Info:
Title: pH-Responsive Micelles From an Oleic Acid Tail and Propionic Acid Heads Dendritic Amphiphile for the Delivery of Antibiotics
Authors: Sifiso S. Makhathini, Calvin A. Omolo, Ramesh Gannimani, Chunderika Mocktar, Thirumala Govender
Year: 2020
Journal: Journal of Pharmaceutical Sciences
DOI: 10.1016/j.xphs.2020.05.011
If you fall off your bike and scrape your knee, or get a paper cut, you probably don’t dwell much on it. You may clean and bandage the wound, but then you go about your day as normal. Just 80 years ago, minor cuts that became infected could be lethal. The use of antibiotics transformed medicine. However, antibiotic resistance, the phenomenon by which bacteria become resistant to drugs, is becoming more common. This resistance develops naturally over time after bacteria are exposed to a drug compound and mutate, causing the drug to no longer be efficacious against the bacteria. This process can be accelerated when drugs do not wipe out an entire bacteria colony because not enough of the drug is delivered to the site of infection. Misuse of antibiotics, such as patients not finishing an entire course of a prescribed drug, is one cause of this. An infamous drug-resistant bacterium is methicillin-resistant Staphylococcus aureus, or MRSA. Severe MRSA infections are usually treated with the drug vancomycin, but some MRSA infections are not vancomycin susceptible.
One way to improve bacterial treatments is to change the way the drug is delivered. Typically, vancomycin is injected into the blood stream and travels around the body. The hope is that the drug will reach the site of infection, but this type of delivery often results in a more dilute concentration of drug than is desired. More extreme doses of vancomycin are not the solution because this can be toxic to our own healthy cells. Therefore, researchers who study drug delivery think of unique and innovative ways to deliver drugs that help the drug effectively reach the site of disease at a desired dosage.
One way that researchers, such as Makhathini et al, achieve this delivery is by using pH-responsive micelles. Micelles are nanostructures that are amphiphilic, meaning they have both a hydrophobic (water-fearing) and hydrophilic (water-loving) segment – think of how amphibians like frogs spend time both in and out of the water. Once at a high enough concentration, the individual amphiphilic molecules will self-assemble into micelles by aligning their hydrophobic groups and their hydrophilic groups (Figure 1). This often creates a sphere-like shape. Researchers have found that drugs can be delivered by encasing the drug molecules inside the micelles, or by adsorption of the drug onto the micelle surface. When a micelle is pH-responsive, it means that the micelle will undergo a rearrangement that is triggered by hydrogen ion concentration. This rearrangement will release drug molecules from the surface or from the inside of the micelle. This pH-responsiveness is particularly useful for antibiotics because a slightly acidic pH is characteristic of many bacterial infections. This allows a higher concentration of the drug to be delivered locally at the infection site, while keeping concentrations in healthy areas of the body at a minimum.
A.………………………………………………………………………………….B.
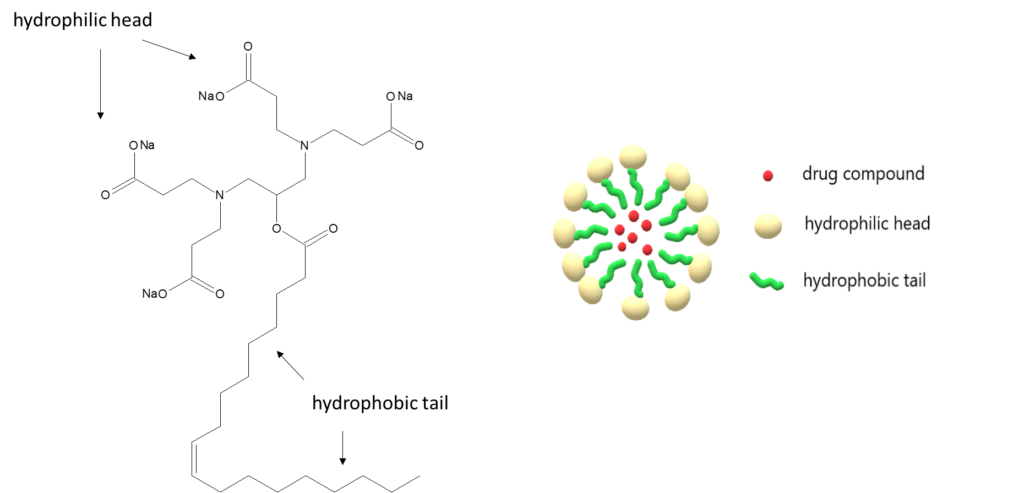
Makhathini et al took the idea of pH-responsive micelles and optimized multiple characteristics of this system to better target MRSA infections. Their system is highly stable once injected, so the micelle won’t degrade once in the bloodstream. Their micelle is also capable of fusing with bacterial cell membranes, helping to target bacterial infections. Because characterizing potential drug compounds is of utmost importance for quality and safety, the research team took a variety of measurements that described the nature of the micelles they created, such as the size of the micelles and the amount of encapsulated vancomycin. An additional biosafety measure the team took was looking at the hemolytic properties of their drug delivery system since it is intended to be administered intravenously. Hemo means blood (just like in hemoglobin or hemophilia), and lysis means to rupture, so if a drug is hemolytic it causes red blood cells to burst. Though their system had a slight hemolytic effect, this effect was lower than similar drug delivery systems reported in the literature.
The team studied the efficacy of vancomycin encapsulated in their micelle vs. bare vancomycin for both S. aureus and MRSA. The encapsulated vancomycin had a lower minimum inhibitory concentration than bare vancomycin for both strains. The minimum inhibitory concentration is the lowest concentration of the drug that stops bacterial growth. Additionally, the encapsulated vancomycin had prolonged activity; its effects lasted 72 hours, whereas bare vancomycin activity could only be observed for up to 24 hours. The encapsulated vancomycin also showed enhanced activity at pH 6.0 vs. pH 7.4, indicating pH-responsiveness. Furthermore, the team used a mice skin infection model and showed that the micelle-delivered vancomycin resulted in a bacterial count that was 8-fold lower than the count from bare vancomycin.
Overall, these differences can be attributed to the micelle encapsulation. Micelle delivery seems to prevent drug molecule degradation, is pH-responsive, and shows enhanced uptake by bacterial cells due to its bacterial membrane-fusing abilities. As antibiotic resistance continues to grow, novel ways to enhance drug delivery will become vital.